Peter Teska, infection prevention application expert, Diversey
Healthcare environmental surfaces and patient care equipment (i.e., surfaces) in healthcare facilities are commonly contaminated with pathogenic microorganisms, so maintaining hygienic surfaces in a healthcare environment is an important part of reducing the risk of healthcare-associated infections (HAIs) for patients receiving care (Otter 2013).
Contamination of surfaces has been shown to impact HAI rates for acute care facilities (Cohen, 2018) (Otter 2013), but manual cleaning and disinfection of surfaces presents certain challenges and has been shown to be inconsistently performed, with cleaning compliance often less than 50% during patient room discharge cleaning (Carling, 2008). Operating room cleaning compliance of surfaces can be even lower, measured at 25% in one study (Jefferson, 2011).
The challenge of maintaining a hygienic patient environment has increased interest in using portable UV-C units to provide an additional level of pathogen reduction on surfaces after the manual disinfection process. The benefits of using these units have been demonstrated in the literature (Boyce, 2011) (Anderson, 2017), but little is known about the long-term risks to healthcare surfaces from repeated exposure to UV-C energy through using these devices.
It is likely that it is necessary to balance applying the dose of UV-C energy necessary to achieve acceptable levels of biocidal efficacy and avoiding excessive UV-C energy that would damage the surfaces being treated.
While many studies demonstrate that UV-C energy can degrade certain polymers, these studies are not reflective of the exposure conditions present in a healthcare facility when using a portable UV-C device. A new study recently accepted for publication investigated this issue using conditions designed to simulate how a portable UV-C device is used in a healthcare facility.
Characterizing surface damage to polymers
Several questions had to be answered to establish the parameters of the study. One of the questions discussed in recent publications (Jo, 2019) (Strader, 2019) is how to properly characterize microscale damage on polymeric surfaces commonly found in a healthcare facility. Microscale surface damage is likely to occur before changes in the bulk mechanical properties of a material can be detected (Jo, 2019) and, thus, detecting when microscale damage has occurred is predictive of early stage surface damage that is likely to be more visible over time through testing more traditional material properties.
Use of a single characterization method has been shown to not be particularly revealing and, consequently, a group of characterization methods has been recommended to detect early onset damage to plastic surfaces (Strader, 2019).
This same approach discussed by Strader (2019) was used to propose a systematic approach to further understand early onset degradation of plastics due to UV-C exposure. Characterization methods for the UV-C study included testing for/using:
- Surface energy (goniometer)
- Optical microscopy (confocal laser scanning microscopy)
- Surface roughness (profilometry/confocal laser scanning microscopy)
- L*A*B color change (colorimetry)
- Whiteness (colorimetry)
- Hardness (nanoindentation)
Materials tested
A range of 10 polymeric surfaces commonly found in healthcare facilities were selected for the testing. The materials included the following:
- Polypropylene (PP)
- Ultra-high molecular weight polyethylene (UHMW PE)
- Polytetrafluoroethylene (PFTE or Teflon)
- Clear polymethyl methacrylate (PMMA or clear acrylic)
- White polymethyl methacrylate (PMMA or white acrylic)
- Polyoxymethylene (Delrin)
- Polyester (polyethylene terephthalate or PET)
- Polycarbonate
- Nylon
- Acrylonitrile butadiene styrene (ABS)
Exposure conditions
The dose of UV-C energy to apply and the exposure conditions governing the dose also had to be established. The literature on testing the biocidal efficacy of portable UV-C units is not consistent in determining the dose needed to achieve a certain level of efficacy or the necessary minimum level of biocidal efficacy that the unit needs to deliver for various reasons, including a lack of a standardized testing method. As there is currently no standardized method for this type of test, the Business and Institutional Furniture Manufacturer’s Association (BIFMA) test conditions were used in this study.
BIFMA (2014) recommends testing for surface damage after exposure to UV-C energy by exposing the surface to a cumulative dose of UV-C energy of 28.8 J/cm2 and then testing for L*A*B color change. The cumulative dose recommended by BIFMA is based on its calculations for using a typical portable UV-C device that treats a surface weekly for seven years and the literature discussing the needed dose to achieve appropriate biocidal efficacy. In the BIFMA method, this cumulative dose is applied in a single exposure, and then the surface is analyzed for color shift to assess whether surface damage has occurred. Additional methods of characterization of surface damage were used for the reasons discussed above.
Results and discussion
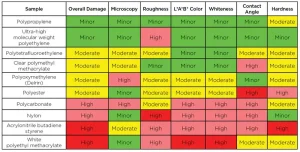
The testing results are summarized in the table, showing the relative surface damage for each characterization technique using arbitrary groupings. The color coding helps show the relative level of damage from low (green) to high (dark red).
The primary implication of the study is that, while it is important to apply an adequate level of UV-C energy to achieve efficacy, it is equally important not to apply excess UV-C energy, as it can accelerate surface damage and prematurely shorten the useful life of the asset for surfaces made from certain polymers. It is possible that excess UV-C energy may not significantly damage an asset made from polypropylene, but it is likely to be a significant factor for an asset made from ABS.
All characterization methods were able to identify one or more specific degradation features from UV-C exposure covering different aspects of physicochemical properties of the surfaces. However, these methods showed different sensitivity and applicability to identify the onset of surface damage. More simply, different characterization methods showed evidence of different types of surface damage, so it is important to test for a range of types of surface damage.
The surfaces that performed the best, PP and UHMW PE, showed only minor evidence of damage across all testing methods.
Four surfaces – ABS, nylon, PC and white acrylic – consistently showed the most evidence of damage across multiple characterization methods. These four surfaces also showed lower amounts of damage across at least one characterization method, demonstrating the importance of using multiple characterization methods. Healthcare facilities may need to consider limiting the treatment of surfaces made from these four plastics to minimize the risk from UV-C exposure.
Summary
Portable UV-C units are beneficial in supplementing existing cleaning and disinfection programs in healthcare facilities, but care must be taken to minimize the excess UV-C energy applied to surfaces, as this can prematurely damage the surface, shortening the useful life of the asset. A lack of standardized methods for UV-C efficacy and surface damage are limiting factors in assessing the suitability of a given UV-C unit. Over time, standardized methods will help healthcare facilities optimize the use of these devices while minimizing potential risks.
Contact: peter.teska@diversey.com
References
- Anderson DJ, Chen LF, Weber DJ, Moehring RW, Lewis SS, Triplett PF, et. al. Enhanced terminal room disinfection and acquisition and infection caused by multidrug-resistant organisms and Clostridium difficile (the Benefits of Enhanced Terminal Room Disinfection study): a cluster-randomised, multicentre, crossover study. Lancet. 2017; 389: 805-814.
- Business and Institutional Furniture Manufacturer’s Association. Health care furniture design – guidelines for cleanability BIFMA HCF 8.1-2014. 2014. Retrieved from: https://cdn.ymaws.com/www.bifma.org/resource/resmgr/standards/BIFMA_CleanGuide_6Oct14.pdf
- Boyce JM, Havill NL, Moore BA. “Terminal decontamination of patient rooms using an automated mobile UV light unit”, Infect Cont and Hosp Epidemiol, 2011; 32 (8): 737-742.
- Carling PC, Parry MF, Von Beheren SM. Identifying opportunities to enhance environmental cleaning in 23 acute care hospitals. Infect Control Hosp Epidemiol. 2008; 29 (1): 1-7.
- Cohen B, Liu J, Cohen AR, Larson E. “Association between healthcare-associated infection and exposure to hospital roommates and previous bed occupants with the same organism”. Infect Cont and Hosp Epidemiol. 2018; 39 (5): 541-546.
- Jefferson J, Whelan R, Dick B, Carling P. A novel technique for identifying opportunities to improve environmental hygiene in the operating room. AORN Journal. 2011; 93 (3): 358-364.
- Jo H, West AM, Teska PJ, Oliver HF, Howarter JA. Assessment of early onset surface damage from accelerated disinfection protocol. Antimicrobial Resistance and Infection Control. 2019; 8:24.
- Otter, et al, “Evidence that contaminated surfaces contribute to the transmission of hospital pathogens and an overview of strategies to address contaminated surfaces in hospital settings”, Am J Infect Control, 2013; 41: S6-S11.
- Strader P, Lee Y, Teska P, Li X, Jones J. Approaches for characterizing surfaces damaged by disinfection in healthcare. Nano LIFE. July 2019; doi: https://doi.org/10.1142/S1793984419500028.