By Ben Sicks, Oksana Gurow and Martin Hessling, Institute of Medical Engineering and Mechatronics of Ulm University of Applied Sciences
With the global trend towards digitalization, the application of touchscreens also is increasing in all areas of life. 1 This includes everyday situations, such as public ticket machines, but also in medical facilities such as hospitals, 2 and is of particular importance here as microorganisms can be transmitted through contact between screen and fingers, potentially leading to the spread of nosocomial infections. In fact, in previous studies, no touchscreen was found to be free of bacteria, even in the medical environment. At a minimum, potentially pathogenic staphylococci were always detected. 3
In principle, it would be possible to treat each screen with chemical disinfectants after every single use, but this would involve a great deal of effort, so this practice is not common.
An earlier study investigated the possibility of automatic touchscreen disinfection by applying UVA radiation or visible violet light. 4 These wavelengths were selected so that the radiation could not endanger the human operator. The experiments were carried out on glass plates contaminated with staphylococci. The radiation from the LEDs was
coupled into the pane from the side to minimize exposure to the user.
A bacterial reduction by more than two orders of magnitude was achieved. However, the time required for a 90% disinfection was at least one hour, which would pose practical limitations in potential future applications.
Another recently much-discussed UV spectral range for disinfection applications is the so-called Far UV-C (200-240 nm). On the one hand, this radiation effectively can destroy the DNA of bacteria, fungi and viruses and thus provides a strong antimicrobial effect. On the other hand, human cells usually are well protected because this radiation is strongly absorbed by proteins, and so, for example, the outer protein-rich dead skin cells in the stratum corneum protect deeper-lying vital cells. 5-13
Therefore, the study presented here aims to investigate the extent to which Far UV-C would be suitable for automatic touchscreen disinfection. Unfortunately, the choice of Far UV-C sources currently is limited. In addition to krypton chloride excimer lamps with a peak emission at 222 nm, which are too bulky to be installed in small devices, there are only a few commercially available Far UV-C-LEDs in the spectral range 230-240 nm with limited radiation powers in the order of only one or a few milliwatts and lifetimes in the order of a few hundred hours.
It is expected that the properties of Far UV-C-LEDs will improve in terms of performance, lifetime and peak wavelength in the future. However, due to the currently low LED outputs, the application of Far UV-C-LEDs for automatic touchscreens only is being investigated for a small quartz plate here, with the quartz plate acting as a highly simplified touchscreen front window / touchscreen model. The irradiation tests are carried out with a staphylococcus strain, as staphylococci are the most common form of touchscreen contamination. 3
Different irradiation approaches are carried out to investigate the impact of Far UV-C radiation on bacterially contaminated quartz plates. Firstly, Far UV-C-LEDs are attached to the side of the quartz plate, which then acts as a light guide. Due to the evanescent field or lack of total reflection in the concept of geometrical optics, bacteria are irradiated over the whole quartz surface without emitting radiation in the direction of the operator. For comparison, the staphylococci also are irradiated conventionally from the top by these LEDs to assess the efficiency of the light guide approach.
Materials and Methods
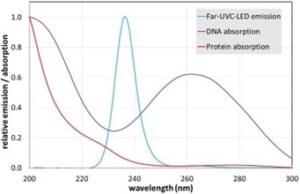
A. Far UV-C LEDs and Experimental Setups
The first irradiation setup was based on four 236 nm LEDs type SF1 (flat lens) from Silanna UV (Pinkenba, Australia). Their emission spectrum is illustrated in Figure 1. The total radiant power at 40 mA was about 1.6 mW per LED, determined with a calibrated photo spectrometer type CAS140D of Instrument Systems (Munich, Germany). The emission angle of the LEDs was 128°.
To implement the first irradiation approach, four of these LEDs were installed at the sides of a quartz pane measuring 50 x 50 x 4 mm3 (Figure 2A), the edges of which were not polished. The LEDs were not positioned in the middle of the edges but slightly shifted to achieve a greater homogeneity and avoid a hotspot in the center of the quartz plate. This quartz plate acted as a light guide with a surface from which the light was reflected, unless the reflection was prevented by microbial contamination, such as bacteria, for example. In the perception of geometrical optics, the light then passed through the bacteria as indicated in Figure 2A.
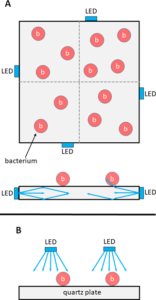
The second setup is schematically presented in Figure 2B, where the quartz plate was conventionally irradiated from the top with the microorganisms on its surface. The irradiation was performed by four of the above-described 236 nm Far UV-C LEDs at a distance of 2 cm. The irradiance in the center of the quartz plane, as determined with the CAS140D spectrometer, was 216 µW/cm2.
B. Test Bacteria and Irradiation Analysis
The selected test organism was Staphylococcus carnosus (S. carnosus), a non-pathogenic staphylococcus strain that exhibits a similar Far UV-C sensitivity as the pathogenic Staphylococcus aureus (S. aureus). 14 S. carnosus was first propagated in tryptic soy extract medium 15 to a concentration of about 108 bacteria/ml, then washed several times in phosphate buffered saline (PBS) and concentrated to 108 bacteria/ml once again.
A home-built spraying device homogeneously contaminated the surface of the quartz plate with the bacterial suspension to be irradiated and another quartz plate that was not irradiated and served as control.
The number of living/surviving bacteria on the irradiated quartz plate was determined after 5, 10, 15, 20, 30 and 60 seconds for the different irradiation set-ups. Additionally, the bacterial concentrations on the unirradiated control quartz plate were determined at the start and end of irradiation.
This microbial assessment was achieved with the aid of eSwabs from Copan (Brescia, Italy). According to the sampling procedure reported by Madsen et al. [16], an area of approx. 25 x 25 mm2 was sampled on the quartz plates using the swab provided, transferred into a destined isotonic solution. The bacteria then were spread on agar plates at various dilution levels. After one day in a 37° C incubator, the surviving bacteria could be determined on the basis of the visible grown colonies. Before irradiation, the quartz plate was divided into four sections (as hinted in Figure 2A) and two opposite quadrants were sampled at each point in time.
To compensate for the limited number of sample areas, the experiments were repeated many times with different irradiation periods. At least three independent experimental runs were carried out for each irradiation duration.
Results
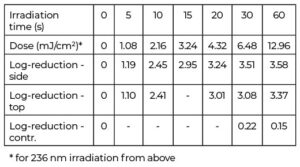
The observed average bacterial reduction is given in a semi-logarithmic representation in Table 1 and Figure 3. In all set-ups, the Far UV-C radiation led to a strong bacterial reduction of about 90% in approximately five seconds and almost three orders of magnitude in 15 seconds, while the unirradiated control revealed no significant decrease in the number of staphylococci.
However, after the initial phase, where the reductions appear to follow exponential curves – straight lines in the half-logarithmic representation in Figure 3 – the bacterial reduction slows down with higher irradiation times and doses, resulting in only marginal further reductions.
Based on the observed reductions after 10 s for 236 nm top surface irradiation and the determined irradiance values, an average log reduction dose of 0.9 mJ/cm2 was calculated for Staphylococcus carnosus.
Discussion and Conclusion
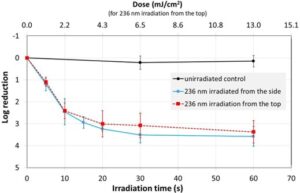
The most important result is that rapid automatic disinfection of small touchscreens using Far UV-C by lateral coupling of the radiation is technically already conceivable even with the low power of today’s Far UV-C-LEDs, as this approach is surprisingly efficient. The total output power of the four 236 nm LEDs of only 6.4 mW was distributed over an area of 25 cm2 of the quartz plate, which formally corresponded to 256 µW/cm2. The disinfection effect achieved was approximately the same or even better as with the 236 nm top irradiation with a real irradiance of 216 µW/cm2, respectively. This observed high efficacy is consistent with the results of our previous investigation on laterally coupled UVA radiation. 4
However, it is worth noting that the current peak wavelength of the Far UV-C-LEDs at 236 nm is not optimal. The ACGIH 236 nm threshold limit values (TLVs) for skin irradiation are approximately 71.5 mJ/cm2 compared to 479 mJ/cm2 at 222 nm. 17 For the aforementioned 236 nm irradiance of 256 µW/cm2, the user should currently only touch the screen for a maximum of about 280 seconds if it does not switch off on contact. At 222 nm, this period would extend to over 30 minutes.
The TLVs for eyes are even lower, but as the eye does not touch the screen and there is practically no irradiation without touching – the total irradiance measured at a distance of only 2 cm already is below 9 µW/cm2 – therefore, the eye is not at risk. Although the present experiments were carried out only with non-pathogenic staphylococci, S. carnosus has a similar Far- UV-C sensitivity to pathogenic S. aureus, including methicillin- resistant S. aureus (MRSA) and other important pathogens such as Pseudomonas aeruginosa. Therefore, this disinfection performance is not limited to (non-pathogenic) staphylococci.
The fast disinfection times, which are almost a factor of 1,000 shorter than in the previously conducted UVA investigation, suggest that in the future, fast, automatic touchscreen disinfection using laterally coupled Far UV-C-LED radiation also is realistic for large screens if the performance and lifetime of these LEDs continues to increase.
Acknowledgement
The authors thank Silanna UV for providing the Far UV-C LEDs and Sila Polat, Jacob Wuchenauer and Luka Zeradjanin for their work on the bacterial contamination system.
This work was funded by the German Federal Ministry of Economics and Technology within the ZIM joint project “Clean Screen” (grant number KK5191602LU1). (Corresponding author: Martin Hessling).
Ben Sicks, Oksana Gurow and Martin Hessling are with the Institute of Medical Engineering and Mechatronics of Ulm University of Applied Sciences (Technische Hochschule Ulm), 89081 Ulm, Germany (email: [email protected]; [email protected]; [email protected]).
References
- Data Bridge Market Research, “Global Touchscreen Display Market, By Screen Types (Capacitive Touchscreens, Resistive Touchscreens, Surface Acoustic Wave Type Displays, Infrared Touchscreens, Others), Application (Personal Use, Professional Use) – Industry Trends and Forecast to 2029,” Data Bridge Market Research, Maharashtra, India, 2022. Accessed: Jan. 30 2023. [Online]. Available: https://www.databridgemarketresearch.com/reports/global-touch-screen-display-market
- Grand View Research, “Commercial Display Market Size, Share & Trends Analysis Report By Product (Digital Signage, Display Monitor, Display TVs), By Technology, By Component, By Display Size, By Display Type, By Application, By Region, And Segment Forecasts, 2020 – 2025,” Grand View Research, San Francisco, USA, 2019. Accessed: Jan. 30 2023. [Online]. Available: https://www.grandviewresearch.com/industry-analysis/commercial-display-market
- M. Hessling, R. Haag, and B. Sicks, “Review of microbial touchscreen contamination for the determination of reasonable ultraviolet disinfection doses,” GMS Hyg. Infect. Control, vol. 16, Doc30, 2021, doi: 10.3205/dgkh000401.
- B. Sicks, A.-M. Gierke, F. Sommerfeld, M. Klein, andM. Hessling, “Disinfection of Transparent Screens by Side-Coupled UVA LED Radiation,” Optics, vol. 4, no. 2, pp. 321–329, 2023, doi: 10.3390/opt4020023.
- J. Glaab et al., “Skin tolerant inactivation of multiresistant pathogens using Far UV-C LEDs,” Scientific Reports, vol. 11, no. 1, p. 14647, 2021, doi: 10.1038/s41598-021-94070-2.
- International Ultraviolet Association, “Far UV-C Radiation: Current State-of Knowledge (White Paper),” Chevy Chase (MD) USA, 2021. Accessed: 2021. [Online]. Available: https://iuva.org/resources/covid-19/Far%20UV-C%20Radiation-%20Current%20State-of%20Knowledge.pdf
- M. Görlitz et al., “Assessing the safety of new germicidal Far UV-C technologies,” Photochemistry and Photobiology, 2023, doi: 10.1111/php.13866.
- M. Hessling, R. Haag, N. Sieber, and P. Vatter, “The impact of Far UV-C radiation (200-230 nm) on pathogens, cells, skin, and eyes – a collection and analysis of a hundred years of data,” GMS Hyg. Infect. Control, vol. 16, Doc07, 2021, doi: 10.3205/DGKH000378.
- E. Eadie, I. M. R. Barnard, S. H. Ibbotson, and K. Wood, “Extreme Exposure to Filtered Far UV-C: A Case Study†,” Photochem. Photobiol., 2021, doi: 10.1111/php.13385.
- D. H. Sliney and B. E. Stuck, “A Need to Revise Human Exposure Limits for Ultraviolet UV-C Radiation†,” Photochem. Photobiol., vol. 97, no. 3, pp. 485–492, 2021, doi: 10.1111/php.13402.
- M. Buonanno, D. Welch, and D. J. Brenner, “Exposure of Human Skin Models to KrCl Excimer Lamps: The Impact of Optical Filtering†,” Photochem. Photobiol., vol. 97, no. 3, pp. 517–523, 2021, doi: 10.1111/php.13383.
- P. Zwicker et al., “Application of 233 nm Far UV-C LEDs for eradication of MRSA and MSSA and risk assessment on skin models,” Scientific Reports, vol. 12, no. 1, p. 2587, 2022, doi: 10.1038/s41598-022-06397-z.
- E. R. Blatchley et al., “Far UV-C radiation: An emerging tool for pandemic control,” Critical Reviews in Environmental Science and Technology, pp. 1–21, 2022, doi: 10.1080/10643389.2022.2084315.
- A.-M. Gierke and M. Hessling, “Investigation on Potential ESKAPE Surrogates for 222 and 254 nm Irradiation Experiments,” Frontiers in Microbiology, vol. 13, p. 942708, 2022, doi: 10.3389/fmicb.2022.942708.
- Deutsche Sammlung von Mikroorganismen und Zellkulturen (DSMZ), M92: trypticase soy yeast extract medium. [Online]. Available: https://www.dsmz.de/microorganisms/medium/pdf/DSMZ_Medium92.pdf (accessed: April 2023).
- A. M. Madsen, H. U. T. Phan, M. Laursen, J. K. White, and K. Uhrbrand, “Evaluation of Methods for Sampling of Staphylococcus aureus and Other Staphylococcus Species from Indoor Surfaces,” Annals of Work Exposures and Health, vol. 64, no. 9, pp. 1020–1034, 2020, doi: 10.1093/annweh/wxaa080.
- 2022 Threshold Limit Values (TLVs) and Biological Exposure Indices (BEIs), American Conference of Governmental Industrial Hygienists (ACGIH), Cincinnati, USA, 2022. [Online]. Available: https://www.techstreet.com/standards/2022-threshold-limit-values-tlvs-and-biological-exposure-indices-beis?product_id=2242171#jumps