Ernest R. Blatchley III, Ph.D., P.E., BCEE, F. ASCE, and Lee A. Rieth, Professor, Lyles School of Civil & Construction Engineering and Division of Environmental & Ecological Engineering, Purdue University
Severe Acute Respiratory Syndrome Coronavirus 2 (SARS-CoV-2) is the cause of coronavirus disease 2019 (COVID-19). The virus was discovered in Wuhan, China, in December 2019. By March 2020, the World Health Organization had characterized the outbreak as a pandemic. The world was unprepared to deal with this virus or the disease it caused. At the time of its discovery, there was little information available to define methods that could be used to control or prevent its transmission.
The costs of the pandemic are still being counted but have been estimated to be on the order of $14 trillion in the US alone. 1 The strongest contributors to these monetary losses were workplace absences, lost sales due to diminished retail shopping, as well as reductions in air travel and public gatherings. But important non-monetary losses were also observed, including more than 7 million deaths, of which more than 1.1 million were reported in the US. There also were important losses in learning and social development among students; the social and economic costs of these conditions will not be known for years to come.
Gradually, the scientific community converged on the understanding that SARS-CoV-2 is transmitted largely through aerosols, which in turn informed control measures. The public health community has made it clear that disease outbreaks, epidemics, and pandemics will occur again. Known pathogens that could contribute to these events include SARS-CoV-2, influenza viruses (including avian or “bird” flu), measles, respiratory syncytial virus (RSV), and human metapneumovirus (HMPV), all of which are respiratory viruses and are spread via similar mechanisms. Beyond these known viral pathogens, we also must be prepared for disease outbreaks that could involve viruses that currently are unknown, as well as other airborne pathogens, including some bacteria.
The collective experiences gained since early 2020 have improved our ability to respond to disease outbreaks, epidemics and pandemics. However, if a pandemic emerged today, it is clear that we are still highly vulnerable to the damage that such a circumstance could bring. In particular, there is very little about our physical infrastructure that has changed since the onset of the COVID-19 pandemic, despite the fact that there is now a clearer understanding of the control measures that are likely to be effective for control of disease transmission, including widespread vaccination, the use of filtering masks and social distancing. These control measures are choices that individuals can make to reduce their risk of contracting disease; in that sense, these measures are relevant at the personal level.
There also are several engineering interventions that are available for control of disease transmission at a community level. From a practical perspective, the technologies that are available and proven to be effective at such a scale include increases in outside air flow into indoor spaces, improvement of air filtration and implementation of germicidal ultraviolet (GUV) devices. In many situations, a combination of these interventions is likely to yield the most robust and effective control of airborne pathogens, but among these technologies, GUV systems offer the greatest potential to reduce human exposure to airborne pathogens, while also presenting the lowest capital and operating expenses, as well as the smallest energy and carbon footprints.
The effectiveness of GUV systems for the control of disease transmission was demonstrated first in the classic study of Wells et al. 2 Many subsequent studies have demonstrated the ability of GUV systems to control transmission of other notable respiratory diseases.
Since these early foundational studies, the technologies that define and support GUV applications have expanded and improved considerably. Important developments have allowed for improved understanding of the spatial distribution of radiant energy in a room where GUV hardware is installed. There also have been critical developments that have improved our understanding of the kinetics of pathogen inactivation; today we know virtually all airborne pathogens are highly susceptible to inactivation by exposure to UV-C radiation. These advances have been accompanied by improvements in lamp technology (as defined by output power, electrical efficiency, and output spectra) and in our understanding of the effects of germicidal UV radiation (generally considered to be coincident with UV-C radiation) on human tissues, especially skin and eyes. These improvements in knowledge and technology allow GUV systems to be installed and operated in a manner that accomplishes effective control of airborne pathogens, while limiting human exposure to below well-established threshold values, such as the Threshold Limit Values (TLVs) defined by the American Conference of Governmental Industrial Hygienists (ACGIH).
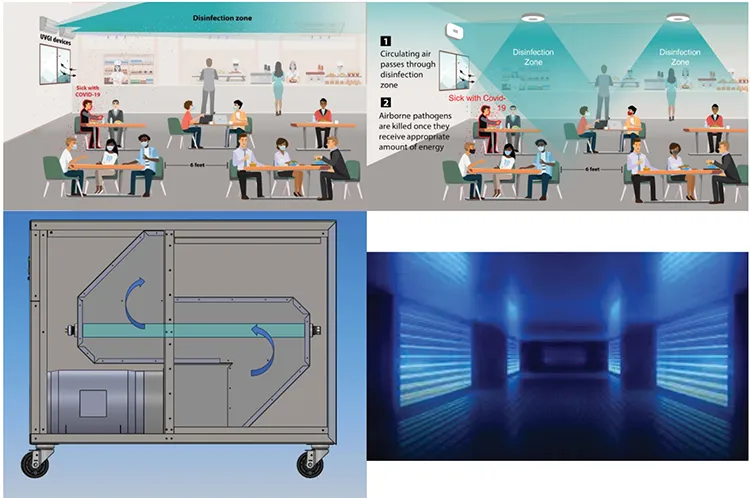
In a broad sense, GUV systems must be implemented in a manner that addresses two somewhat conflicting design objectives. First, the system must deliver sufficient UV-C radiation to accomplish effective inactivation of airborne pathogens. Second, human exposure must be maintained below the TLVs. There are several GUV configurations that allow these design objectives to be met. The most common of these are so-called “upper-room” (UR) GUV systems, wherein GUV lamps are housed within a fixture that includes (parabolic) reflective surfaces and louvers to direct lamp output in a nominally-horizontal direction. Typically, UR-GUV fixtures are mounted on a wall such that their output is confined to the upper portion of the air space in a room, well above the heads of room occupants (see Figure 1). UR-GUV systems commonly involve low-pressure mercury lamps as the source of UV-C radiation; these systems are characterized by output that is essentially monochromatic, with a characteristic wavelength of 254 nm. However, with recent improvements in output and efficiency of UV light-emitting diodes (UV LEDs), UR-GUV systems are being developed based on UV LEDs. These devices are characterized by polychromatic output with spectral peaks that typically are observed in the range 260-280 nm.
An important emerging technology involves the use of Far UV-C radiation, informally defined as radiation with wavelengths in the range of 200-230 nm. The most common source of Far UV-C radiation is the optically-filtered krypton chloride excimer (KrCl*) lamp, which is characterized by nearly monochromatic output centered at approximately 222 nm. Far UV-C radiation has been demonstrated to be highly effective for inactivation of pathogens. However, because Far UV-C is absorbed strongly by proteins and other critical biomolecules, it has very limited ability to penetrate human skin and eye tissues. As a result, Far UV-C offers very limited potential to cause damage to these tissues. In turn, this allows humans to be exposed to substantially higher doses of Far UV-C than from conventional UV-C sources, such as low-pressure mercury lamps or UV LEDs. By extension, this allows so-called “whole-room” (WR) GUV configurations to be implemented. WR-GUV systems typically involve ceiling-mounted fixtures that house optically-filtered KrCl* lamps. A schematic illustration of a WR-GUV system is presented in Figure 1.
Human exposure can be effectively eliminated by placing the GUV source inside an opaque chamber. Two common GUV configurations that involve this approach include GUV-based air cleaners and in-duct systems. GUV-based air cleaners typically involve one or more GUV lamps housed within a cabinet. A fan is used to force air through the irradiated zone within the cabinet; the cabinet may also be fitted with one or more air filters. In-duct systems involve placement of GUV lamps within the ductwork of an existing heating, ventilation and air conditioning (HVAC) system. Illustrations of a GUV-based air cleaner and an in-duct system are presented in Figure 1.
Despite considerable practical experience and recent advances, several barriers to the implementation of GUV systems remain. Among these are a lack of standards for design, testing and validation of GUV systems. Several formal and informal groups are working to develop such standards. Considerable attention has been given to these issues in presentations at recent high-profile conferences, including the IUVA Americas Conference, 7 the 2nd International Congress on Far-UVC Science and Technology 8 and Indoor Air 2024. 9
At a more fundamental level, there are considerable gaps in understanding the behavior and characteristics of GUV systems. These gaps exist among the general public but are also common within the engineering and scientific communities. An important example is fear of exposure to GUV radiation. Some of this fear stems from the common understanding that exposure to GUV radiation can lead to skin and eye tissue damage, with endpoints that include erythema (sunburn), photokeratitis (aka “snow blindness” or “welder’s flash”) and skin cancer. All of these are well-established medical conditions that are related to exposure to solar UV radiation. It is important to recognize that the solar UV radiation we experience at earth’s surface is entirely within the UV-A and UV-B portions of the electromagnetic spectrum. The reactions that are promoted by GUV (aka, UV-C) radiation are similar to those that are promoted by UV-B radiation, but GUV radiation has a much lower ability to penetrate human skin and eye tissues than either UV-B or UV-A. Moreover, there is a firm understanding of the wavelength-dependence of UV-induced damage (i.e., action spectra) for human skin and eyes. This understanding informs installation of GUV systems that are safe for humans, as quantified by the TLVs.
More recently, there have been reports of adverse effects of GUV radiation on indoor air chemistry. This behavior is largely associated with the formation and decay of ozone. GUV at wavelengths below roughly 242 nm can promote ozone formation by photolysis of molecular oxygen. Ozone is an irritant of the human respiratory system and eyes, so its presence in indoor air needs to be controlled. Far UV-C lamps at 222 nm are known to produce ozone; however, they are relatively inefficient ozone generators and the ozone they produce is easily mitigated through the use of proper air ventilation. In most situations, the amount of ozone we are exposed to in an indoor setting is more strongly influenced by outdoor ozone that is brought indoors through an HVAC system than production by a Far UV-C source.
A related issue is UV-induced decay of ozone, which is promoted by all GUV wavelengths. Photodecay of ozone leads to formation of reactive intermediates which, through secondary reactions, can lead to formation of ultrafine particles. These particles can penetrate deep into lung tissue and may lead to respiratory problems. Current knowledge indicates that ozone formation and decay, as well as the secondary reactions that lead to ultrafine particle formation, are important problems that require research. However, it appears that the effects of these processes on indoor air quality can be minimized by the use of proper ventilation and perhaps air filtration.
As is the case with other applications of UV, such as disinfection of water, the existence of remaining open questions does not (or should not) prevent the application of GUV systems, which have a well-established ability to yield public health improvements. In a broad sense, we need to consider the benefits and costs of these systems as they relate to human health. Given the potential for GUV systems to control airborne pathogen exposure, as well as the certainty that we will face future disease outbreaks, epidemics and pandemics, it is prudent to consider how we might promote the installation of GUV systems in public settings. Moreover, the community of engineers and scientists who work on indoor air quality need to continue to pursue research to address the open questions that remain about GUV systems.
References
- Reference found at https://healthpolicy.usc.edu/article/covid-19s-total-cost-to-the-economy-in-us-will-reach-14-trillion-by-end-of-2023-new-research/
- Wells WF, Wells MW, Wilder TS. The environmental control of epidemic contagion I: an epidemiologic study of radiant disinfection of air in day schools. Am J Hyg 1942;35:97-121
- Image from: https://www.cdc.gov/coronavirus/2019-ncov/community/ventilation/GUV.html
- Image from: https://forum.effectivealtruism.org/posts/2rD6nLqw5Z3dyD5me/does-the-us-public-support-ultraviolet-germicidal
- Image modified from Roman Fountains, Inc.
- Image from: https://www.agcoombs.com.au/news-and-publications/advisory-notes/ultraviolet-germicidal-irradiation-for-hvac-applications/
- IUVA Americas Conference, Orlando, FL 20-22 May 2024
- ICFUST, St. Andrews, Scotland 18-21 June 2024
- Indoor Air 2024, Honolulu, HI 7-11 July 2024