By M. Clark, R. Zuber and M. Ribnitzky, Gigahertz-Optik
The potential of UV-C ultraviolet light in combating the spread of COVID-19 has resulted in an increase in demand for conventional technologies, such as low-pressure Hg lamps (254 nm), as well as many rapid developments in UV-C LED (260 to 280 nm) devices and products. A significant limitation is that UV radiation is both carcinogenic and cataractogenic. Far UV-C radiation (< 240 nm) is of particular interest as it is able to inactivate pathogens whilst being less harmful to skin. Far UV-C is absorbed strongly by biological materials and whilst it does penetrate viruses and bacteria, it does not penetrate the outer layers of dead human skin.
The accurate measurement of any UV source, especially Far UV-C, places great demands on equipment and the techniques employed. Radiometers/dosimeters, typically used for assessing UV-C installations, require a good cosine-weighted spatial response and should be calibrated using a source that has a similar spectral distribution to the sources to be measured. Comprehensive UV lamp and UV LED device characterization requires the use of UV-optimized spectroradiometers. The causes and elimination of internal stray light effects need to be understood and implemented; otherwise, they will introduce significant errors. This may result in unnecessarily failing devices when making photobiological safety assessments.
UV-C germicidal applications
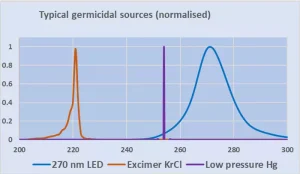
UV-C radiation has been widely used for germicidal applications for many years and already was gaining wider interest prior to COVID-19 as a means of combating antibiotic resistance and healthcare-acquired infections. Advances in UV-C LED technology and research into the use of Far UV-C are providing many opportunities for innovation that all require reliable radiometry in order to establish

germicidal efficacy (Chart 1) as well as photobiological safety (Chart 2). Various standards and regulations exist with respect to photobiological safety, but germicidal efficacy mostly is reliant on published research – most of which refers to the use of 254 nm radiation as produced by low-pressure mercury lamps.
Irradiance
Both efficacy and safety require the measurement of irradiance in terms of incident power per area in order to establish dose over the duration of exposure – one Joule per square meter being one Watt-second per square meter. Note that irradiance is proportional to the cosine of the angle of incidence.
Strictly speaking, for airborne pathogens, the authors should be referring to fluence and fluence rate rather than irradiance and dose, but the units of measure are the same. Fluence rate refers to the irradiance from all angles that is incident on a small region of space.
Germicidal UV applications require the measurement of irradiance in order to establish dose. The unit of irradiance is W / m2. Dose measured in J / m2 where 1 J = 1 Ws.
Surface disinfection: irradiance (mW / cm2) / dose (mJ / cm2)
Air disinfection: fluence (mW / cm2) / fluence rate (mJ / cm2)
UV radiometers
Practical measurements generally are made as a planar irradiance measurement using a UV radiometer. Depending on the source configuration and environment, it may be necessary for fluence rates to be estimated from planar measurements taken in several directions at a single point, and it may be appropriate to use UV reflectance data to aid modelling (Image 1). There are many UV radiometers available, but achieving accurate, meaningful measurements with good inter-instrument agreement is much more difficult than might be expected.
In principle, UV radiometers are relatively simple devices comprising a cosine diffuser, normally with some optical filtering to match the response, with a photodetector that’s connected to a suitable amplifier and display electronics. The device needs to be calibrated against some reference source.
There are a number of quality indicators that determine the measurement accuracy – or measurement uncertainty, to use a better term – that can be achieved with a UV radiometer. The three issues of primary concern are spatial response, spectral response and calibration. Also of relevance, but of secondary importance, typically are such matters as temperature effects, stability, linearity, fatigue, etc.
Spatial response
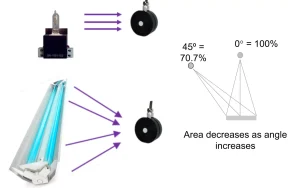
Just because a device has some opaque white diffuse-looking sensor, it doesn’t mean it has a good cosine response in the Far UV-C. Germicidal UV applications frequently require measurements over very wide angles so a poor cosine response easily can become the dominant source of error (Image 2). Note that most manufacturers don’t calibrate devices with an extended source.

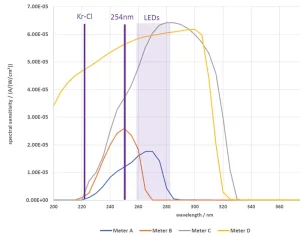
Spectral response
An ideal UV-C radiometer will have a perfectly flat in-band spectral response, completely block longer wavelengths and have an infinitely sharp cut-off (Chart 3) – not possible in reality, of course. In Chart 4, spectra responsivity of four high-quality UV radiometers is shown. Provided they are appropriately calibrated, all are quite suitable for measuring low-pressure mercury lamps.
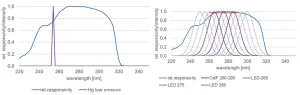
Calibration
Because of their non-flat spectral responsivity, UV radiometers ideally should be calibrated using a source that has a similar spectral distribution to the sources to be measured. In practice, most UV-C radiometers are just calibrated using a 254 nm source, which can result in significant errors when measuring UV-C LEDs or excimer sources. A radiometer that incorporates calibrations for multiple source types is advantageous (Chart 5).
Calibrations should be done in accordance with, and traceable to, international standards such as those established by the International Organization for Standardization (ISO), International Laboratory Accreditation Cooperation Mutual Recognition Arrangement (ILAC MRA) or Deutsche Akkreditierungsstelle (DAkkS). Look out for suppliers being a little creative with their use of the terms “traceable” and “accredited” when it comes to calibration.
Photobiological safety
Various photobiological safety standards exist, with IEC 62471 being a widely applied product standard. Most standards incorporate the long-established ICNIRP weighting function for actinic UV, and a daily limit of 30 J/m2 often is referred to. Note that this weighting function varies by around four orders of magnitude in little more than the bandwidth of a typical UV LED (Chart 6). Standards either specify or permit measurements to be performed with an 80-degree field of view due to the limited field of view of the eye and because little obliquely incident light penetrates to epidermal skin tissue.
ACGIH 2022
This year, the ACGIH have made changes to the actinic UV hazard function in the far UV-C region. This creates opportunity for Far UV-C germicidal sources to significantly increase their germicidal effectiveness whilst operating at photobiologically safe levels. However, in the case of 222 nm excimer lamps it’s necessary to look carefully at the longer wavelength UV-C radiation produced in addition to the 222 nm peak (Chart 7).
KR-Cl filter

To reap the full benefit of an excimer lamp’s 222 nm emission, it’s essential that a suitable filter is fitted to block the longer wavelength UV-C emissions (Chart 8).
In order to establish photobiologically safe operation, high dynamic range UV spectroradiometric measurements are required. Double monochromator-based systems are considered to be the reference standard for such measurements, but these are large, slow-scanning laboratory instruments and often can’t be used conveniently. Array-based spectrometers offer many advantages such as speed, size and ease of use and are therefore most widely used. However, it’s essential that any array-based UV spectroradiometer demonstrates measurement results comparable to double monochromator systems. In particular, this refers to their internal stray light rejection.
Stray light: Causes and reduction techniques
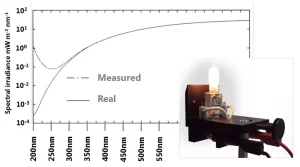
When performing spectroradiometric measurements in the UV region, internal stray light generally is the fundamental limiting factor of measurement capability and huge errors can result as shown in Chart 9. There are various causes of stray light within a spectrometer including multiple orders of diffraction, scattering from optical surfaces, 0th order reflections, etc. All contribute to stray light being seen by the detector.

A number of techniques can be employed to reduce internal stray light. Good optical design with optimized components such as a holographic grating and high-quality mirror surfaces are an important starting point. Long-pass and band-pass filters are particularly useful for reducing stray light effects resulting from out-of-range wavelengths. A tunable laser can be used to characterize a spectrometer at any in-range wavelength which enables a stray light correction matrix to be applied to subsequent measurements (Chart 10). Careful implementation of these combined stray light correction techniques does make possible double monochromator-like performance, but very few commercially available instruments achieve this.
Summary
In summary, there are a few key points:
- Most UV-C radiometers really are suitable only for use with low-pressure mercury sources, but a few commercial devices are available with a spectral response that covers the Far UV-C range required for excimer sources. However, it’s important they’re calibrated with a source having a similar spectral distribution.
- A good cosine response is essential when measuring the extended sources typically found in germicidal UV applications.
- Devices often have some low-level responsivity to ambient lighting, which can be to the detriment of photobiological safety measurements.
- Far UV-C research and hazard measurements are best undertaken with spectroradiometers, but it’s essential that they have excellent internal stray light performance. Most array spectrometers claiming UV coverage don’t offer this.
- 222 nm excimer lamps require a suitable filter for optimum balance between germicidal efficacy and photobiological safety.
Mike Clark, BSc MIET, is business development manager for Gigahertz. He has worked on the development, application and marketing of optical radiation measurement equipment for more than 35 years.
Dr. Ralf Zuber is a physicist who focused on optical technologies during his studies. For about 10 years, he has been working at Gigahertz-Optik GmbH, Germany, first as a product manager and, for the past seven years, as head of R&D. In his work, he develops a variety of optical measurement devices, along with carrying out active research in spectroradiometers. He is an active member of several Technical Committees of CIE Division 2 and also is active in the DIN standardization committee for light. For more information, email [email protected] or visit www.gigahertz-optik.de.