by James P. Malley, Jr., Ph.D., University of New Hampshire, Durham, New Hampshire
The risk of contracting or spreading COVID-19 has sparked increased interest in the application of UV technologies for air disinfection. Knowledge on the use of upper room air UV disinfection technologies in healthcare facilities has been accumulated from decades of its demonstrated effectiveness in combating tuberculosis. Interest and use of air disinfection UV technologies ranging from conventional low-pressure mercury vapor lamp systems emitting primarily at 254 nm to UV LEDs with typical emissions in the 265 to 295 nm range and far UV-C technologies emitting primarily at 222 nm are all growing.
Once air disinfection technology is selected, designed and installed in accordance with technical specifications, its long-term effectiveness for providing public health protection depends exclusively on proper operation and maintenance. This article addresses lamp aging and fouling, lamp cleaning and the potential benefits of monitoring/sensors to confirm UV dose.
UV technology systems for air: Monitoring
The largest body of knowledge on lamp aging is found in the low-pressure mercury vapor lamp technologies as summarized in numerous publications including the US Environmental Protection Agency’s UVDGM published in 2006.¹ General advice from that experience suggests typical lamp lives on the order of 8,000 hours with a 20 percent loss in UV-C output by the end of lamp life. The overall range from this large database shows lamp lives for some poorly operated systems can be as low as 4,000 hours or as high as 10,000 hours in fully optimized systems. Two major caveats on this range are the number of on-off cycles a lamp is subjected to during a typical 24-hour operating period and the overall power quality in terms of brownouts or power surges in the system. The greater the on-off cycling, the shorter the lamp life.
The UVDGM also points out the benefits and the vital role a UV lamp sensor can play to ensure proper lamp operation and maintenance practices. Data for other UV lamp systems are far less comprehensive given their relatively new and/or limited use. In UV LED systems, research suggests the higher drive currents (e.g., 700 mA vs. 350 mA) will result in significant degradation of UV-C output in the first 1,000 hours of operation. The literature on Far UV-C indicates that it is typically generated by lamps containing a mixture of krypton and a halogen gas resulting in most of the UV-C energy in the 200 nm, 207 nm, 222 nm and 254 nm range. The two commercially available and common sources are KrCl and KrBr. The KrCl gas discharge typically emits 90% of its UV radiation around 222 nm, and a couple percent at 258 nm. Filters typically are aimed at eliminating the 258 nm radiation. KrBr gas discharge lamps emit primarily at 207 nm but are not as efficient as 222 nm lamps. Neither of these produce much 200 nm, but the KrCl does produce a small amount of energy at 195nm. Commercial applications of Far UV-C sources often recommend optical filters that result in essentially monochromatic light at 222 nm. This source then is optically filtered and produces an essentially monochromatic UV-C at 222 nm, which has produced very promising research results and sparked widespread interest and potential applications. This filtered lamp source has a limited track record so lamp life is difficult to predict, but initial studies suggest it will be stable for at least 4,000 hours and may demonstrate longer life similar to the conventional low-pressure mercury vapor lamps.
One final point about lamp life is to remind the users that many UV systems for air do not operate continuously. Thus, lamp life, and the actual device operating time before the need for lamp changes, can be significantly different and vary as much as tenfold.
An equally valuable resource for understanding maintenance of upper room air UVGI systems was published by USAID’s End Tuberculosis Transmission (ETT) Initiative in 2017.³ This source is highly recommended for operators of room air UV disinfection systems. It advises: “Upper-room GUV fixtures will vary based on make, model and manufacturer. Care must be taken to follow all manufacturers’ recommendations for installation, maintenance and repair. There are, however, standard or universal maintenance procedures that need to be done to maintain the efficiency and effectiveness of the fixtures. A visual inspection wearing appropriate eye protection should be performed periodically, and, if a lamp failure is observed, corrective action should be taken immediately to return the fixture to service. Major tasks discussed include: Emission (fixture output) performance and safety measurements; fixture and lamp cleaning; routine inspections; repairs and replacement; disposal/cleanup of nonfunctional lamps, ballasts, fixtures.”
The literature also is clear on the importance of UV monitoring using a calibrated radiometer for two purposes: 1) to ensure that exposures are all well within the latest health and safety guidelines (many sources quote the ACGIH recommendation of not exceeding an eight-hour exposure limit for a 254 nm UV dose of 6 mJ/cm2; and 2) ensuring that the air UV disinfection unit is operating properly and delivering the specific public health protection. A useful rule of thumb provided by USAID 2017 for 254 nm UV wavelengths states, “The cornea of the eye is the most sensitive organ to GUV over exposure; therefore, for lower room safety, in most cases eye-level irradiance should be no more than 0.4 μW/cm2 at 1.7 m above the finished floor anywhere in the room.” UV dose guidelines to ensure safety at different wavelengths are published in many sources, including a detailed summary of many key aspects of UVGI published by Kowalski in 2009.4
Most of the air UVGI literature is based on the availability of qualified maintenance and monitoring staff either employed by the owner or hired as a service provider to the owner. This approach was logical since using UV technologies for controlling airborne transmission of disease has been a commercial endeavor practiced by hospitals, owners or operators of commercial HVAC systems. However, as the numbers and use of these technologies grow and encompass smaller or individual consumer-based markets, the need to equip these devices with either UV wipers to keep lamps and reflective surfaces clean and/or UV sensors or monitors to trigger cleaning events will be vital to ensure safe and effective operation.
If sensors are chosen, they should meet specifications previously established for the water industry as summarized in the 2006 USEPA UVDGM. The sensors will inform the user when maintenance is required, when lamp replacement is needed, and ensure that an appropriate and safe UV dose is being delivered to the air by the device.
There have been significant improvements in all types of sensor technologies, including the monitoring of UV systems emitting either monochromatic or polychromatic wavelengths. This, combined with the growth of commercial and consumer applications of IoT, allows monitoring and control of UV disinfection technologies for room air through typical devices such as smartphones.
UV technology systems for air: Cleaning
The when and how of UV system cleaning will be a function of the manufacturer’s specifications and operations manual, as well as the application (location) of the device.
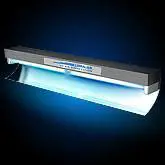
Numerous sources suggest that monitoring data be developed to determine the optimal cleaning frequency. Site-specific data suggests that the frequency of cleaning needed can vary by a factor of three from dirty environments to cleaner environments. UV technology systems for air typically involve the lamps, the reflective surfaces and may have other components such as housing or louvers that require cleaning. Fouling of these surfaces occurs due to the presence of smoke, dust and haze in the air (also known as aerosols) as explained in the classic research and textbook of S.K. Friedlander in 1977.5 The literature for UVGI applied to air recommends a maximum period between cleanings for dirty environments of three months and for cleaner environments of six months.
With the onset of the COVID-19 pandemic and increased speculation on the use of UV technology systems for air, an ongoing experiment was initiated by the MalleyCAT UV research group at UNH in early June 2020.
The experiment was designed to examine the rate of lamp fouling using a conventional upper room UVGI system (Figure 1) placed in a restaurant setting on July 1, 2020. The three-lamp system was installed in what was considered a worst-case location (impacted by the kitchen and clean-up areas) of a local restaurant, and the hours of system operations were monitored continuously. The UV irradiance at 254 nm was monitored at the beginning and end of each day with a calibrated, NIST-traceable IL1700 radiometer.
The preliminary experimental results are shown in Figure 2. These data have been fully QA/QC’d, but have not been peer-reviewed. These data suggest that significant fouling occurs, that rates of fouling can be highly variable, that fouling will reach a steady state level without cleaning events and that recovery of the UV lamp irradiance after cleaning events is about 95% with the loss likely due to lamp aging.

Visual inspection during the cleaning events found that lamps and reflector surfaces were fouled with a combination of dust particles and an “oily-watery” mixture. Cleaning events followed the procedures detailed in the USAID, 2017. The experimental data also has been subject to changing restaurant operating behaviors (normal inside table service, socially distanced inside table service and no inside table service) that can have a confounding effect.
The takeaway recommendations from these preliminary data are that cleaning frequency in these particular circumstances may need to be weekly and the value of a continuously recording, IoT networked, sensor on this UV system would provide valuable guidance on performance and the need for maintenance.
Author’s Note: A few sources were reviewed and included throughout the article. Their findings, advice and current UNH laboratory data were combined to provide this article.
Contact: James P. Malley, Jr., Ph.D., [email protected]
References
- USEPA UVDGM nepis.epa.gov/Exe/ZyNET.exe/600006T3.TXT
- iuva.org/resources/covid-19/
- USAID, End Tuberculosis Transmission (ETT) Initiative, 2017. www.stoptb.org/wg/ett/assets/documents/MaintenanceManual.pdf
- Kowalski, 2009. link.springer.com/book/10.1007/978-3-642-01999-9
- S.K. Friedlander, 1977. aiche.onlinelibrary.wiley.com/doi/epdf/10.1002/aic.690230526