N.D. LaTourette
environmental engineer
H.R. Roakes
project manager, Sanborn, Head and Associates, Inc.
J.P. Malley, Jr.
editor-in-chief, UV Solutions
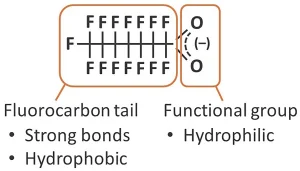
Per- and polyfluoroalkyl substances (PFAS) are a family of roughly 5,000 human-made chemicals that have been used in commercial and industrial applications since the 1940s. PFAS are made of carbon-fluorine chains with attached functional groups that often give the chemicals surfactant qualities, meaning they are partially hydrophobic and partially hydrophilic. The carbon-fluorine chain is also very resistant to degradation. Due to those properties and their abundant use, they are highly prevalent and persistent in the environment and in human blood. Studies have shown that PFAS in drinking water, including perfluorooctanoic acid (PFOA) and perfluorooctane sulfonic acid (PFOS), may pose a risk to human health.
Human health concerns
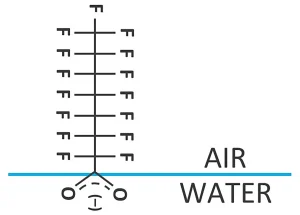
PFAS were first detected in the blood and serum of occupationally exposed workers in the 1970s, but there was limited awareness of PFAS abundance in the environment and potential human health threats until the 2000s1. PFAS, particularly perfluoroalkyl acids (PFAAs), are commonly found in human blood, regardless of occupation, due to their prevalence in consumer goods, their ability to bond easily to blood proteins and their long half-lives in humans1. Although humans are potentially exposed to a wide range of PFAS through consumer goods and the environment, most research has largely been focused on PFOA, PFOS and some other PFAAs.
Several laboratory animal epidemiological studies have indicated that exposure to PFAS may result in adverse health effects. Epidemiological studies have found increased risk of thyroid disease, increased blood cholesterol levels, decreased response to vaccines, decreased fertility in women, increased risk of high blood pressure and preeclampsia (high blood pressure and fluid retention) and lower infant birth weight2,3,4. Animal studies suggest PFAS exposure is linked to immune system and liver damage as well as birth defects, delayed development and newborn deaths2. The International Agency for Research on Cancer concluded in 2016 that PFOA is possibly carcinogenic to humans, and more study is needed. The US Environmental Protection Agency also has reported that PFOA and PFOS are potentially carcinogenic, although non-cancer risk is the driver of the current EPA Lifetime Health Advisory (LHA) level for PFOA and PFOS3.
Health advisories, standards and regulation
PFAS are not directly regulated under the Resource Conservation and Recovery Act (RCRA), the Clean Water Act or the Clean Air Act and are not included as a Comprehensive Environmental Response, Compensation, and Liability Act (CERCLA) hazardous substance, like many regulated contaminants3. However, through CERCLA, federal regulators may require response actions to protect public health and the environment, as well as to comply with other requirements (e.g., state promulgated standards). In 2016, the EPA released an LHA of 70 ng/L for a combined concentration of PFOA and PFOS in drinking water4. The LHA was calculated to offer a margin of protection for pregnant and nursing women, as their children are the most sensitive to exposure3. This LHA provides guidelines for water suppliers; however, it is a non-enforceable advisory. A 2016 data analysis of the EPA’s Unregulated Contaminant Monitoring Rule (UCMR) program data, which consisted largely of public water supplies serving greater than 10,000 people, found that over six million Americans’ drinking water supplies had levels of PFOA and PFOS above the LHA6. Since the UCMR sampling, many public water supply systems have taken measures to reduce PFOA and PFOS levels in their drinking water3.
Since the LHA was issued in 2016, various state regulatory agencies have developed more stringent health advisories for drinking water and ground water. Additionally, some states have proposed or promulgated drinking water Maximum Contaminant Limits (MCLs) for PFAS. For instance, New Hampshire has proposed MCLs for PFOA, PFOS, perfluorohexanesulfonic acid (PFHxS) and perfluorononanoic acid (PFNA) of 12, 15, 18 and 11 parts per trillion (ppt) respectively5. These proposed standards, along with many of the other proposed or promulgated state MCLs, are among the most conservative limits to date.
Treatment technologies
Due to their physical and chemical properties, including strong carbon-fluorine bonds, multiple ionic states, variable carbon chain length and differing functional groups, PFAS are difficult to treat with current technologies6. These physical properties result in general resistance to oxidation and reduction reactions, biological recalcitrance, negligible volatility and thermal resistance, which do not allow for many common treatment mechanisms to be effective7.
In terms of UV technologies, preliminary research suggests that PFAS typically found in wastewater treatment plants are not affected by the typical UV technologies and doses employed for wastewater disinfection. Similarly, the high UV doses and the resulting radical oxidation pathways employed by UV AOP technologies have not been effective against the PFAS typically found in contaminated ground waters and drinking waters. AOPs have been used to oxidize PFAS precursors, resulting in a net increase in more stable PFAS (e.g., PFOA, PFOS). A similar mechanism is used in the total oxidizable precursor assay (TOP assay) laboratory method for measuring precursor PFAS.
Due to widespread detection of PFAS in the environment and increasing public attention due to their persistence and bioaccumulation, there is a very fertile environment for additional research on PFAS and UV technologies. The need for innovative photolytic treatments or coupled photo-chemical or photo-biological techniques should continue to be explored by UV technology researchers. Biological processes, such as ozone bio-filtration, which may show promise for treating PFAS, also will benefit from post UV disinfection to control bacterial counts.
Proven adsorption liquid treatment methods
Granular activated carbon (GAC) is the most used PFAS water treatment method, particularly for private and public drinking water systems8. GAC removes PFAS from water by sorbing the PFAS onto its surface, primarily via a hydrophobic interaction between the activated carbon media and the carbon chain of the PFAS. Greater than 90% removal can be achieved for most effluents, with greater than 99% removal or greater for longer-chain PFAS8. This is because shorter-chain PFAS typically break through GAC vessels faster than longer-chain PFAS. Due to this, using GAC to treat for shorter-chain PFAS can be more costly. Removal efficiencies are specific to GAC media, operations and water chemistry, so pilot studies should be carried out prior to full-scale design to estimate applicability and breakthrough times. Both virgin and reactivated GAC are available and can show similar treatment results7. After use, GAC requires transport offsite for reactivation, destruction or disposal.
Ion exchange (IX) resins are another common and proven adsorption treatment method for removing PFAS from water. IX resins adsorb PFAS via electrostatic interactions between the negatively charged ionic PFAS functional groups and positively charged exchange sites on the resins8. Hydrophobic interactions may also occur between the organic or plastic polymers of the resin and the carbon-chain of the PFAS.
IX resins typically have higher adsorption capacities and faster reaction kinetics compared to GAC8, enabling typically smaller filter vessels than for GAC. IX resins may be either single use, which require offsite destruction or disposal, or regenerable. Regenerable IX resins can be regenerated on-site via chemical and/or brine flushes, although the regenerate solution then needs to be managed.
Developing adsorption treatment technologies
Biochar and molecularly imprinted polymers are also being studied as potential PFAS treatment technologies. Biochar is a carbon-rich, porous solid synthesized from biomass such as wood products6. Biochar generally has a large surface area to volume ratio and is a potential alternative to GAC, but variability in its physical and chemical properties make consistent PFAS removal difficult. Biochar is also not currently able to be regenerated6. Molecularly imprinted polymers are polymers designed with surface charges that are selective for specific compounds. PFAS removal performance of imprinted polymers is being further studied7.
Membrane filtration technologies
Reverse osmosis has shown greater than 99% removal of PFAS from bench- and pilot-scale studies at DWTPs and WWTPs, and nanofiltration has demonstrated 90% to 95% removal efficiencies in lab tests for PFAS. The better performance of reverse osmosis is due to the smaller pore size. However, there are significant concerns regarding fouling8. Additionally, both reverse osmosis and nanofiltration require significant energy requirements to push the water through membranes. Management and treatment of the highly concentrated rejectate is also a concern.
Developing coagulation technologies
Electrocoagulation is another developing technology that uses positively charged metal ions (often zinc or iron) released from an anode, and negatively charged hydroxyl groups generated from the water and a cathode to remove PFAS from water9,10. When the metal ions interact with the hydroxyl groups, they form flocs, which then electrostatically sorb PFAS9. The floc then settles out and can be removed from the solution.
This is a treatment technology that has only been researched at the bench scale; however, it has shown removal rates exceeding 95% for certain PFAAs9. Like the other methods described above, the largest concern is management and treatment of the concentrated PFAS-containing sediment.
Developing fraction technologies
Fractionation using ozone works by allowing micro- to nano-sized ozone bubbles (10 to 10,000 nm) to travel up through a reactor column. The ozone bubbles react with the water that surrounds them; hydroxyl radicals (OH-) form around the surface11. The charges on hydroxyl radicals electrostatically interact with PFAS and carry them to the top of the reactor column, where they generate a froth or foam, which aids in removing the compounds11,12. The foam then is captured and destroyed7. This technology has been implemented at full scale in Australia by consulting firm Arcadis. Following the full-scale fractionation process in Australia, the effluent is treated using nanofiltration. Fractionation with air has also been considered as a potential treatment technology11. However, peer-reviewed studies of these treatment methods were not found.
Developing destructive technologies
Destructive technologies have and are continuing to be studied at bench scale. Most of them employ oxidative manipulation techniques of the water containing PFAS8. A variety of peer-reviewed articles detail the specifics of the following treatment methods, but due to their early phase of development, only the basics are described below.
Electrochemical oxidation applies high voltage electricity to the water via electrodes (titanium-oxide and boron-doped diamond have shown the best results)13,14. Oxidative radicals are generated in the water from the high voltage and remove electrons from PFAS13. Theoretically, this process will occur until the PFAS species are mineralized. This process often uses an additional oxidative chemical solution to increase oxidative conditions in solution15. Using mesh electrodes, this technology has the potential to be used in-situ.
Other forms of electrochemical oxidation research have used combinations of UV light at high doses, hydrogen peroxide addition and/or ozonation to create radical advanced oxidation conditions15. Again, the destruction of PFAS is via an “electron-stripping” mechanism due to the oxidative conditions of the water. Sonochemical degradation uses ultrasound and oxidative chemicals to generate oxidative radicals in water15. The destruction mechanism is also via the removal of electrons from PFAS. Plasma uses high voltage electricity exclusively to generate plasma, a highly conductive and reactive state of matter. When there is sufficient contact between the plasma and PFAS, the plasma has the ability to remove electrons from the PFAS.
Additionally, microbial degradation mechanisms have been studied. Acidimicrobium species Strain A6 and pseudomonas species have shown the ability to degrade PFOA and PFOS16. Advanced reduction processes using aqueous iodide have also been investigated15. Besides the generally very high energy requirements for destructive treatment technologies, a main concern is that they may create more mobile and toxic by-products, or they may mobilize or transform co-contaminates present in the water8.
Contact: N.D. LaTourette, [email protected]; H.R. Roakes, [email protected]; J.P. Malley, Jr., [email protected]
References
- Interstate Technology and Regulatory Council, “History and Use of Per- and Polyfluoroalkyl Substances,” November 2017. [Online]. Available: https://pfas-1.itrcweb.org/wp-content/uploads/2017/11/pfas_fact_sheet_history_and_use__11_13_17.pdf. [Accessed 26 September 2019].
- United States Environmental Protection Agency, “Fact Sheet: Draft Toxicity Assessments for GenX Chemicals and PFBS,” November 2018. [Online]. Available: https://www.epa.gov/sites/production/files/2018-11/documents/factsheet_pfbs-genx-toxicity_values_11.14.2018.pdf. [Accessed 13 December 2019].
- Interstate Technology and Regulatory Council, “Aqueous Film-Forming Foam (AFFF),” October 2018. [Online]. Available: https://pfas-1.itrcweb.org/wp-content/uploads/2019/03/pfas-fact-sheet-afff-10-3-18.pdf. [Accessed 13 December 2019].
- United States Environmental Protection Agency, “Fact Sheet: PFOA & PFOS Drinking Water Health Advisories,” November 2016. [Online]. Available: https://www.epa.gov/sites/production/files/2016-06/documents/drinkingwaterhealthadvisories_pfoa_pfos_updated_5.31.16.pdf. [Accessed 3 October 2019].
- New Hampshire Department of Environmental Services, “NHDES Proposes New PFAS Drinking Water Standards,” 28 June 2019. [Online]. Available: https://www.des.nh.gov/media/pr/2019/20190628-pfas-standards.htm. [Accessed 13 October 2019].
- Interstate Technology and Regulatory Council, “Environmental Fate and Transport for Per- and Polyfluoroalkyl Substances,” March 2018. [Online]. Available: https://pfas-1.itrcweb.org/wp-content/uploads/2018/03/pfas_fact_sheet_fate_and_transport__3_16_18.pdf. [Accessed 26 September 2019].
- A. Corey Theriault, “Water Treatment Technologies for PFAS: Current and Next Generations,” in The 35th Annual International Conference on Soils, Sediments, Water and Energy, Amhurst, Massachusetts, 2019.
- Interstate Technology and Regulatory Council, “Remediation Technologies and Methods for Per- and Polyfluoroalkyl Substances (PFAS),” March 2018. [Online]. Available: https://pfas-1.itrcweb.org/wp-content/uploads/2018/03/pfas_fact_sheet_remediation_3_15_18.pdf. [Accessed 26 September 2019].
- Y. Wang, H. Lin, F. Jin, J. Niu, J. Zhao, Y. Bi and Y. Li, “Electrocoagulation mechanism of perfluorooctanoate (PFOA) on a zinc anode: Influence of cathodes and anions,” Scince of the Total Environment, no. 557-558, pp. 542-550, 2016.
- B. Yang, Y. Han, G. Yu, Q. Zhuo, S. Deng, J. Wu and P. Zhang, “Efficient removal of perfluoroalkly acids (PFAAs) from aqueous solution by electrocoagulation using iron electrode,” Chemical Engineering Journal, no. 303, pp. 384-390, 2016.
- X. Dai, Z. Xie, B. Dorian, S. Gray and J. Zhang, “Comparative study of PFAS treatment by UV, UV/ozone, and fractionations with air and ozonated air,” Environmental Science Water Research & Technology, no. 5, pp. 1897-1907, 2019.
- J. Mcdonough, “First Time Use of Ozofractionation to Treat AFFF Release and Validation by Top Assay,” in New England water Environment Association, 2019.
- T. X. H. Le, H. Haflich, A. D. Shah and B. P. Chaplin, “Energy-Efficient Electrochemical Oxidation of Perfluoralkly Substances Using a Ti4O7 Reactive Electrochemical Membrane Anode,” Environmental Science & Technology, no. 6, pp. 504-510, 2019.
- A. M. Trautmann, H. Schell, K. R. Schmidt, K. M. Mangold and A. Tiehm, “Electrochemical degradation of perfluoroalkyl and polyfluoroalkyl substances (PFASs) in groundwater,” Water Science & Technology, vol. 10, no. 71, pp. 1569-1575, 2015.
- N. Merino, Y. Qu, R. A. Deeb, E. L. Hawley, M. R. Hoffmann and S. Mahendra, “Degradation and Removal ethods for Perfluoroalkyl and Polyfluoroalkyl Substances in Water,” Environmental Engineering Science, vol. 33, pp. 615-649, 2016.
- S. Huang and P. R. Jaffe, “Defluorination of Perfluorooctanoic Acid (PFOA) and Perfluorooctance Sulfonate (PFOS) by Acidimicrobium sp. Strain A6,” Environmental Science & Technology, vol. 53, pp. 11410-11419, 2019.