Steve Reinecke
clinical advisor and VP of international sales, Proximity Systems, Inc.
Nosocomial pathogens, commonly referred to as healthcare-associated infections (HAIs), cause life-threatening complications for patients and costly consequences for healthcare facilities. One in 31 patients hospitalized in the United States in 2017 had at least one HAI on any given day1. In a public health report, an estimated 1.7 million people a year acquire an HAI during a hospital stay, costing the healthcare industry $35.7 billion to $45 billion annually2,3.
High-touch surfaces are a source of HAIs that cause secondary transmissions of bacteria, directly through patient contact or indirectly through the hands of healthcare workers who touched a contaminated surface4. Infectious pathogens, including Clostridium difficile (C. diff), vancomycin-resistant Enterococci (VRE), methicillin-resistant Staphylococcus aureus (MRSA), Acinetobacter baumannii, Pseudomonas aeruginosa and norovirus can contaminate high-touch surfaces, survive disinfection attempts, transfer to the hands of healthcare workers and infect patients upon contact5.
Multiple studies have demonstrated how frequently touched surfaces can contain pathogens. A study in a medical ICU found colonization rates were higher for keyboards in rooms with patients positive for MRSA, and another study found keyboards tested positive for the growth of two or more microorganisms, including coagulase-negative Staphylococci (100% of keyboards), diphtheroids (80%), Micrococcus species (72%), Bacillus species (64%) and oxacillin-resistant Staphylococcus aureus (4%)6,7.
In addition to keyboards in patient rooms, portable medical equipment (PME) and computers on carts – also known as workstations on wheels (WOWs) – can be used hundreds of times a day, acting as a mobile reservoir for multidrug-resistant microorganisms. The top three most common interactions involving PME and patients were WOW to patient (22.6% of total sequences), patient to WOW (20.4%) and patient to IV pump (16.1%), demonstrating that frequently touched PME and WOWs are potential sources of contamination from patients or the environment8. Pathogens can spread from WOW to patient and patient to WOW, as established in a study that revealed daily cleaning of WOWs was nonexistent over a baseline evaluation period9.
If a health system’s cleaning and disinfection protocols are insufficient, harmful microorganisms can be transmitted, underscoring the need for an extensive review aimed at improving cleaning and disinfection techniques10.
UV-C
A way to expand cleaning protocols is with the advent of ultraviolet (UV) disinfection devices. Used as early as 1878 by Arthur Downes and Thomas P. Blunt, the short wavelength light was investigated to sterilize bacteria11. Since then, UV light has been used in air and water treatment and as a surface disinfectant of fruit and vegetables12. The short wavelength of UV, UV-C (between 250 and 280 nm), is considered germicidal and can inactivate bacteria, viruses and other microorganisms by damaging their deoxyribonucleic acid (DNA) to prevent the spread of HAIs13,14.
New no-touch decontamination technologies can offer benefits for disinfecting high-touch surfaces in a healthcare environment, such as in-room computer workstations, WOWs and PME. A recent article reported that ultraviolet light disinfection is “successful in reducing the bio burden of a room and [has] been shown to stop outbreaks associated with environmental contamination”15. Multiple studies also have demonstrated the effectiveness of UV light to reduce HAIs. Specifically no-touch methods, including UV devices, have confirmed their ability to reduce HAIs on high-touch surfaces16.
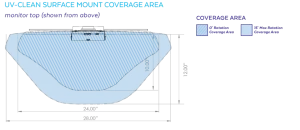
Point-of-care UV units, such as UV-CLEAN (Proximity Systems, Tomball, Texas), are attached to or positioned above high-touch surfaces (Figure 1). The unit provides automated cleaning cycles of UV-C light to inactivate microorganisms at the genetic level by damaging their DNA. A built-in motion sensor enables the unit to safely emit UV-C light to disinfect when in-room workstations, stationary equipment, WOWs or PME are not in use and no motion is detected. The following clinical study evaluated the effectiveness of UV-CLEAN in a hospital setting.
Materials and methods
Location
In an effort to reduce cross-contamination of HAIs in its facility, HCA Houston Healthcare Southeast partnered with Proximity Systems to complete a study of the use an automated UV-C disinfection unit in the neurology unit and Intermediate Medical Care Unit (IMCU) at HCA Houston Healthcare Southeast, a fully accredited, 345-bed medical facility located in Pasadena, Texas, from March 2019 to April 2019.
The study involved 52 computer workstations. Of these workstations, 16 were Ergotron StyleView mobile computing carts (two different models) that were primarily used and stored in the hallways. There were also 36 wall-mounted Proximity Systems workstations (two different models) in 36 individual patient rooms. Of the 36 rooms, 32 were occupied, and the other four rooms had been occupied within the past 24 hours of initial swabbing.
Collection of baseline data
Baseline keyboard cultures were taken before UV exposure of all 52 workstations using the COPAN ESwab 480c Collection Kit. Using sterile saline, sterile gauze was moistened, and the swab was wetted using an aseptic technique to prevent cross-contamination. Keys A-Z were swabbed by rolling the swab over the surface of each key. The entire surface of the spacebar and enter key were also sampled. A single swab was used for each workstation.
Negative controls were processed at the beginning, during and end of the sampling process using a wet sterile gauze with the saline solution to ensure aseptic conditions were maintained throughout the sampling process. Samples were sent to Lodestar Diagnostic Laboratory in Houston, Texas. Samples were plated on Blood Agar and Rose A66 aerobically and Chocolate Agar anaerobically. Catalase and Staphtex testing were performed on all suspected Staphylococcus aureus and all positives were tested for Cefoxitin sensitivity. VRA screening was using PYR and vancomycin sensitivity.
Installation of UV disinfection units
After samples were obtained, the UV-CLEAN disinfection unit was installed. Both cart models had sliding keyboard trays that stored under the work surface when not in use and pulled out to the front of the work surface when in use. Because of the difference in cart models, two different locations were chosen to mount the unit. Additionally, 10 carts were older models that offered minimal space between the keyboard and bottom of its work surface, resulting in the development of a custom bracket designed to mount the unit to the back of the keyboard storage area. Six of the second cart type had sufficient space to use the standard keyboard mount stand supplied with the unit, allowing the unit to be positioned three inches above the back of the keyboard, directly over the function keys.
There were two different models of wall-mounted workstations, consisting of a flip-down work surface, which holds a keyboard and mouse. When the work surface is down, a monitor is revealed, and the keyboard and mouse are 20 inches from the UV-C light disinfection unit. When the work surface is closed, the keyboard and mouse are still exposed to UV-C light. Fourteen of the workstations allowed for the use of the standard retrofit configuration that features a bracket to mount the UV-CLEAN unit to a shelf directly above the monitor. Twenty-two units used the standard surface-mount configuration that attached the unit to the top of a monitor. Both solutions were approximately 20 inches from the work surface where the keyboard was placed, allowing for a large area of exposure to UV-C light.
Device settings
At the time of the testing, the units were set up in the following preset time configuration:
- Clean time (the period the device is producing UV-C light): 300 seconds (five minutes).
- No motion time (the length of time the device will allow to pass before producing UV-C after the motion sensor has communicated an absence of movement): 60 seconds (one minute).
- Wait time (downtime scheduled between cleaning cycles that are unrelated to motion sensor activity): 60 minutes (one hour).
Collection of post-disinfection data
After two weeks of usage, identical samples were taken from carts and wall-mounted units using the identical sampling procedure (one wall-mounted unit was removed from the study as no access was allowed by the collectors due to an airborne isolation protocol patient being in the room). As with the pre-disinfection samples, post-disinfection samples were sent to Lodestar Diagnostic Laboratory in Houston, Texas.
Results
Comparison of computer workstation bioburden at baseline vs. post-UV light disinfection
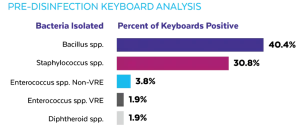
Initial culturing of the computer workstation keyboards prior to the installation of UV-CLEAN units identified bacteria in 75% of the units. Of the 16 mobile computing carts, seven (44%) were found to have bacteria on the surface of the keyboard. Of the 35 wall-mounted units, 31 (89%) were found to have bacteria on the surface of the keyboard. Wall-mounted units in three patient rooms had multiple bacteria isolated. A variety of bacteria was isolated on the mobile computing carts and wall-mounted computer workstations as shown in Figure 2.
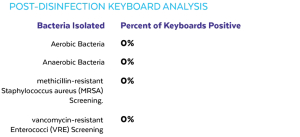
The post-UV light disinfection cultures were sent for evaluation of the effectiveness of the units. All samples came back negative for growth on all surfaces swabbed, indicating a 100% reduction in keyboard bioburden, as shown in Figure 3. All negative controls taken during the study were negative for bacterial growth.
Internal audit data
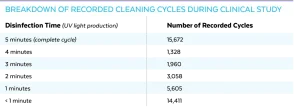
The UV-CLEAN unit has an internal audit feature that records when the unit disinfected and when the unit registered motion. During the 14-day study, the 51 computer workstations recorded 42,034 cycles with 15,672 being complete, uninterrupted 300-second cleaning cycles, as shown in Table 1.
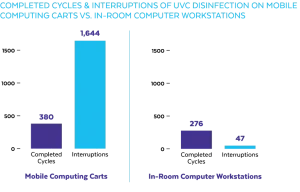
During the study, the mobile computing carts saw significantly more activity than did the in-room computer workstations (Fig 4A, 4B). It was observed during the study that the motion sensor on the carts was being triggered by activity other than the use of the keyboard.
UV-C exposure
Using high-speed photography, it was determined that the maximum UV-C exposure obtained prior to the motion sensor triggering the bulb to turn off was 30 µW/cm2. Total exposure time never exceeded one second. The National Institute for Occupational Safety and Health (NIOSH) recommends that the time of exposure to an intensity of 100 microwatts per sq. cm. at wavelength 254 nm not exceed one minute; and based on the NIOSH recommendation, the maximum time to be exposed of 30 µW/cm2 should not exceed 200 seconds during an eight-hour period17.
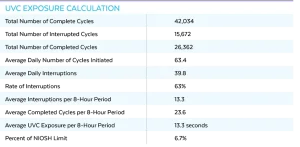
As noted previously, the settings for the unit during this study was five minutes of cleaning time per hour or after a one-minute period of inactivity after motion is detected. The data from the more than 42,034 records was analyzed, and 63% of cleanings were interrupted. This resulted in 13.3 interruptions on average per eight-hour shift. Based on the exposure testing done, this would give 13.3 seconds of 30 µW/cm2, resulting in just under 7% of the maximum dosage recommendation set by the NIOSH for an eight-hour shift (Table 2).
Discussion
The need for hand washing protocols and chemical disinfection on high-touch surfaces in healthcare environments has been long recognized; however, studies have shown cleaning regimens are not always followed. Healthcare workers touch many surfaces daily, resulting in the need for consistent hand hygiene, including use of hand sanitizer before and after interaction with patients, workstations or PME. Although considered a standard practice, hand hygiene compliance throughout the US is only 40%, which is why daily disinfection of frequently touched surfaces is also a standard procedure critical for reducing HAIs18,19.
When hand hygiene is followed consistently, as was the case in this study with healthcare workers using mobile computing carts, high-touch surfaces like keyboards have less chance of contamination. During the initial culturing, there was a lesser percentage of mobile computing carts that tested positive for bacteria than the wall-mounted workstations in patient rooms. Hospital protocol was to sanitize hands prior to entering and after leaving a patient’s room. Since mobile computing carts were seldom brought into a patient room, and nurses sanitized their hands after leaving a patient’s room and prior to touching the keyboard, the keyboard had less chance of contamination than the wall-mounted workstations in a patient’s room.
Also vital to reduce microbial contamination is environmental cleaning, as described as the physical act of cleaning a surface followed by an application of a disinfectant. Yet data from a recent study proves not all disinfecting agents are implemented correctly in relation to dwell time and type of surface – both impacting their effectiveness20.
Infectious pathogens can spread if disinfection measures are inconsistent, which is why an automated cleaning modality is an effective complement to existing cleaning protocols. When used as a supplemental strategy, UV light can enhance disinfection and decrease bioburden to decrease HAI rates21. This study found that UV-CLEAN, an automated, low-intensity UV-C radiation point-of-care unit, was effective at eliminating harmful pathogens on keyboards attached to WOWs and wall-mounted workstations.
When used in conjunction with existing cleaning protocols, the unit’s timed disinfection cycles also mitigated human error, a contributing factor to the spread of infection within a hospital. Because the unit is small (6″ x 1″ diameter) and available in multiple configurations, it also can be installed on other PME, including pumps and imaging machines, that may or may not be cleaned prior to or after patient use.
This study also found that the UV-C light motion sensor was being triggered by activity other than use of the keyboard. To mitigate this, the no-motion time default setting on the UV-CLEAN unit was changed from one minute to four minutes post-study. The data suggests this change would significantly decrease the amount of cycling and extend the bulb life but not impact the effectiveness of the UV disinfection. Based on that change and using the UV-C exposure data collected, the exposure limit would decrease to 3% of the maximum dosage recommendation set by the NIOSH for an eight-hour shift.
Conclusions
While increased access to information at the bedside has proved to deliver better patient outcomes, it has also introduced greater risk for patients to acquire an HAI. This study demonstrates the efficacy of UV-CLEAN as an automated UV disinfection unit, reducing bacterial burden on high-touch surfaces in and out of a patient’s room.
When used as a complement to existing cleaning protocols, UV-CLEAN can safely target high-touch surfaces with no disruption to patient care or staff workflow and provide an audit to ensure disinfection is taking place.
As previously published on InfectionControl.tips, June 26, 2019 https://infectioncontrol.tips/2019/06/26/evaluating-a-no-touch-uvc/
Contact: Steve Reinecke, sreinecke@proximitysystems.com
References
- 2017 national and state healthcare-associated infections progress report. Center for Disease Control and Prevention https://www.cdc.gov/hai/pdfs/progress-report/2017-Progress-Report-Executive-Summary-H.pdf. Accessed April 29, 2019.
- Klevens RM, Edwards JR, Richards CL Jr, et al. Estimating health care-associated infections and deaths in U.S. hospitals, 2002. Public Health Rep. 2007;122(2):160–66.
- Scott II RD. The direct medical costs of healthcare-associated infections in U.S. hospitals and the benefits of prevention. Center for Disease Control and Prevention 2009;7.
- Rutala WA, Weber DJ. Guideline for Disinfection and Sterilization in Healthcare Facilities. Washington, DC: Centers for Disease Control (U.S.) 2008 (Updated 2017);11-12.
- Otter JA, Yezli S, French GL. The role played by contaminated surfaces in the transmission of nosocomial pathogens. Infect Control Hosp Epidemiol 2011;32:687-99.
- Bures S, Fishbain JT, Uyehara CF, Parker JM, Berg BW. Computer keyboards and faucet handles as reservoirs of nosocomial pathogens in the intensive care unit. Am J Infect Control. 2000;28:465-71.
- Rutala WA, White MS, Gergen MF, Weber DJ. Bacterial Contamination of Keyboards: Efficacy and Functional Impact of Disinfectants. Infect Control Hosp Epidemiol 2006;27(4):372-77.
- Jinadatha C, Villamaria FC, Coppin JD, et al. Interaction of healthcare worker hands and portable medical equipment: a sequence analysis to show potential transmission opportunities. BMC Infectious Diseases. 2017;17.
- Po JL, Burke R, Sulis C, Carling PC. Dangerous cows: An analysis of disinfection cleaning of computer keyboards on wheels. Am J Infect Control. 2009;37(9):778-80.
- Dubberke ER, Carling P, Carrico R, Donskey, CJ, Loo, VG, McDonald, LC, et al. Strategies to prevent clostridium difficile infections in acute care hospitals: 2014 Update. Infection Control & Hospital Epidemiology. 2014;35(6):628-645.
- Downes A, Blunt TP. IV. On the influence of light upon protoplasm. Proceedings of the Royal Society of London. 1879;28:199-12.
- Cutler TD, Zimmerman JJ. Ultraviolet irradiation and the mechanisms underlying its inactivation of infectious agents. Anim Health Res Rev. 2011;12(1):15-23.
- Meulemans C. The basic principles of UV–disinfection of water. The J of the International Ozone Association. 1987;9(4):299-313.
- Reed NG. The history of ultraviolet germicidal irradiation for air disinfection. Public Health Rep. 2010;125(1):15–27.
- Carrico RM, Bryant K, Lessa F, Limbago B, Fauerbach LL, Marx JF, et al. Guide to Preventing Clostridium Difficile Infections. Association for Professionals in Infection Control and Epidemiology, Inc. (APIC); 2013:61.
- Weber DJ, Kanamori H, Rutala WA. ‘No touch’ technologies for environmental decontamination. Current Opinion in Infectious Diseases. 2016;29(4):424-431.
- Kowalski W. Ultraviolet Germicidal Irradiation Handbook. 2009:297 (Table 12.2-293-299).
- Association for Professionals in Infection Control and Epidemiology (APIC). Overview: Infection control prevention and control for computers in patient care areas. Preventing Infection in Ambulatory Care. 2011.
- Kundrapu S, Sunkesula V, Jury LA, Sitzlar BM, Donskey CJ. Daily Disinfection of High-Touch Surfaces in Isolation Rooms to Reduce Contamination of Healthcare Workers Hands. Infection Control & Hospital Epidemiology. 2012;33(10):1039-42.
- Han JH, Sullivan N, Leas BF, Pegues DA, Kaczmarek JL, Umscheid CA. Cleaning hospital room surfaces to prevent health care-associated infections: a technical brief. Ann Intern Med. 2015;163(8):598–07.
- Dancer SJ. Controlling hospital-acquired infection: focus on the role of the environment and new technologies for decontamination. Clin Microbiol Rev. 2014;27(4):665–690.