By Carlton Gibson, UV design and technical support manager, Sensor Electronic Technology, Inc.
Introduction
Ultraviolet Photocatalytic Oxidation (UVPCO) has been shown to be effective at removing VOCs in small scale demonstrations since the 1990s.6 It has been suggested as a potential solution for energy savings by reducing the required air changes per hour in buildings and all the associated costs with treating that air.3 While a wide range of UV sources may be used to stimulate the reaction, UV-A LED systems offer several advantages as compared to traditional UV-A sources.
With <1% wall-plug efficiency (WPE), incandescent black lights are not an economical choice for UVPCO applications. Gas-discharge lamps may achieve as high as 30% WPE in UV wavelengths, but UV-A LEDs on the market today have several times longer lifetime and roughly double the WPE. Also, the added benefits of no ozone production, no lifetime drop for power cycling, no hazardous waster, instant on-off and higher mechanical sturdiness are all ways UV-A LED adoption in this technology can drive down the cost.
Recently, UVPCO technology has been shown to have disinfection efficacy against airborne microorganisms like Phi X 174 – a common surrogate for SARS-CoV-2 viral testing.2 In the past, UV LED supplier Seoul Viosys (hereafter: UV LED supplier) has analyzed the efficacy of small-scale UV-A LEDs in PCO systems by analyzing various wavelengths, air flow rates, irradiance levels and photocatalyst surface area. Now, it looks to further develop this technology by combining UV-C and UV-A LED sources to improve air purification applications by means of dual-disinfection mechanisms and increased UV exposure on the surface of TiO2 photocatalytic filters.
Using more UV sources achieves the added benefit of increased irradiance uniformity on the surfaces of the photocatalytic material. However, this comes at the expense of higher system price and potential material compatibility challenges. While LPM lamps still are cheaper and more energy efficient than UV-C LEDs and the lifetime improvement isn’t quite as impressive as UV-A LEDs, the other benefits of LEDs listed still apply.
Experimental Setup and Test Methods
The first experiment, a study of bacterial inactivation as a function of UV-A and UV-C dosage combinations, was conducted by the UV LED supplier’s internal laboratory. The lab is BSL-2 certified and tests to KOUVA AS-01 standards but is not accredited. The experiment was performed using a nebulizer to introduce bacteria containing droplets to a 400 L fixed volume of air in the test chamber. Bacterial concentrations in CFU/m3 were measured by collecting multiple agar plate samples at each test time – exposed to the chamber air via samplers. Previous testing had shown negligible decay rates within the time frame of the test, so concentrations were compared with time 0 vs. a control system operating in parallel.
This experiment had several trial groups. The first had two 365 nm LEDs outputting 300 mW of radiant flux each with 120° viewing angle, which were positioned 20 mm away from a TiO2 coated ceramic photocatalytic filter (PCF) that had dimensions of 75 mm x 25 mm x 8 mm. For the next trial, the same system was tested, but with a single, 10 mW 275 nm LED of the same viewing angle and working distance positioned in between the two UV-A LEDs. Finally, the UV-A LEDs and PCF were removed to better understand the benefit added by the separate mechanism of DNA absorption of UV-C photons.
An additional larger-scale experiment was performed with the virus Phi X 174 as the target microorganism. This testing was performed by Korea Testing Laboratory (KTL). This lab is IEC 17025 and 17065 certified by KAS. Testing was done on a UV-C air sterilization system using similar nebulizing and sampling technology in a 60 m3 volume of air. The system utilized 16 UV-C LEDs at 10 mW of radiant flux each. Built-in fans circulated the air through the UV-C chamber at a 45.8 m3/min air flow rate and operated for 30 minutes total.
Results
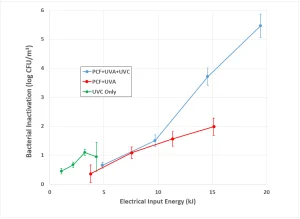
In Figure 2, it can be seen that the combination of mechanisms in the first experiment provides a significant benefit for a small addition of input energy. After two hours of recirculation, the UV-A+PCF mechanism yielded roughly 2 log (99%) reduction of S. aureus. The UV-C treatment yielded slightly less than 1 log (90%) reduction in the same time. But, when combined together, the results exceeded 5 log (99.999%) reduction.
The second experiment showed that a 2 log (99%) reduction of airborne virus Phi X 174 could be achieved in 30 minutes of operating time in a large chamber volume.
Discussion
The difference between the PCF + UV-A line and the PCF + UV-A + UV-C line represents the addition of two mechanisms (PCO from UV-C and UV-C germicidal irradiation). The exact ratio of how much disinfection efficacy comes from each mechanism cannot be precisely determined from this data, but as the UV-C radiant flux is small, it is expected that the PCO effects of UV-C on the PCF is small in comparison. After the first log reduction, the hybrid combination of these two technologies showed higher log reductions in the same amount of time than either could achieve alone. This could be an indicator of more energy-efficient systems to be developed in the future. In terms of raw energy consumption, the UV-C system alone outperformed the UVPCO system for reduction of microorganisms, but UV-C alone will do little for gas removal. More research is necessary to find an optimal balance between UV-C and UV-A energy in a UVPCO system to maintain suitable levels of VOC reduction and maximize microorganism reduction.
ASHRAE Position Document on Filtration and Air Cleaning 2021 notes, that while there are no studies available regarding direct health effects of PCO equipment indoors, there are concerns about PCO systems’ health effects. Some studies showed an increase in certain VOCs – possibly related to the presence of human bioeffluent alcohols and air flow rates higher than the treatment system being tested could handle.1,4 When combining UVPCO systems with UV-C for disinfection purposes, one should take care that sufficient photocatalytic material is present and that appropriate flow rates are selected to minimize the chance of incomplete oxidation reactions occurring.
As mentioned before, some challenges exist when adding UV-C LEDs to finished products, as UV-C light has a more drastic degrading effect on polymers than UV-A. Over time, in the presence of UV-C, plastics may discolor, become more brittle and develop a powdery substance on the surface. This challenge can be overcome by utilizing polymers with UV inhibitors or using metal in the vicinity of the UV sources.
UV-C LEDs also are more expensive than UV-A LEDs – meaning the initial cost to scale up this kind of system is more challenging. However, steady performance increases and wider adoption of UV-C LEDs are expected to drive the cost down in the near future, while optimizations reduce the time to pay back the initial investment.
Conclusions
The combination of these two technologies may be a driving force for cleaner, cheaper indoor air. The removal of VOCs, odors, viruses and bacteria is highly beneficial, and the use of LEDs enables a sustainable, environmentally friendly solution.
Carlton Gibson is the UV design and technical support manager for Sensor Electronic Technology, Inc. For more information, email [email protected].
References
- ASHRAE Position Document on Filtration and Air Cleaning. https://www.ashrae.org/file%20library/about/position%20documents/filtration-and-air-cleaning-pd-feb.2.2021.pdf.
- Chun-Chieh Tseng & Chih-Shan Li (2005) Inactivation of Virus-Containing Aerosols by Ultraviolet Germicidal Irradiation, Aerosol Science and Technology, 39:12, 1136-1142, DOI: 10.1080/02786820500428575
- Fisk W.J., Seppanen O., Faulkner D., and Huang J. (2005) Economic benefits of an economizer system: energy savings and reduced sick leave. ASHRAE Transactions 111(2). LBNL-54475.
- Hodgson, A.T., H. Destaillats, D.P. Sullivan, and W.J. Fisk. 2007. Performance of ultraviolet photocatalytic oxidation for indoor air cleaning applications. Indoor Air 17:305–16.
- Jensen, M.M. (1964). Inactivation of Airborne Viruses by Ultraviolet Irradiation, Appl. Microbiol. 12:418–420.
- Obee T.N., and Brown R.T. (1995) TiO2 Photocatalysis for indoor air applications: Effects of humidity and trace contaminant levels on the oxidation rates of formaldehyde, toluene, and 1,3-butadiene. Environ. Sci. Technol. 29:1223-12316